Deep into the cosmic abyss lies an intriguing aspect of the universe – the divide between what we can observe and what we cannot. This fascinating dichotomy, known as the Observable versus the Unobservable Universe, has astrophysicists and cosmologists on the edge of their seats. This exploration is not just about gazing at distant galaxies, but rather peeling back the layers of the universe to understand its intricate design and workings.
In this exploration, we will delve into the concept of the Observable Universe, its parameters, and how it is determined by the speed of light and the age of the universe. Further, we will confront the enigma of the Unobservable Universe, the part of the cosmos that remains unseen and untouched by human knowledge, yet plays a pivotal role in our understanding of the universe’s origin, structure, and fate.
Lastly, we’ll look at how modern science, aided by cutting-edge technology, is striving to unravel the mysteries shrouded in the cosmic beyond. By bridging the gap between the Observable and Unobservable Universe, we hope to gain new insights into the universe’s incredible depth and complexity. So buckle up for an exciting journey to the furthest reaches of the cosmos, beyond the limits of our current understanding! 🌌🔭
The Observable Universe: A Closer Look
In the pursuit of understanding the universe, astronomers have divided it into two main categories: the observable and the unobservable universe. The observable universe is a term that refers to the part of the universe that can be observed from Earth. It’s a sphere with Earth at its center and extends about 93 billion light years in all directions.
The radius of the observable universe is determined by the distance that light has traveled since the Big Bang, around 13.8 billion years ago. However, due to the expansion of the universe, the current distance to the edge of the observable universe is about 46 billion light years.
This observable universe is populated by galaxies, stars, and other celestial bodies that emit light or other electromagnetic radiation that can be detected with current technology. It also includes matter that can be observed indirectly, such as dark matter.
Understanding the Cosmological Principle
At the heart of modern cosmology lies a simple but powerful idea known as the Cosmological Principle. This principle posits that on a sufficiently large scale, the universe is both homogeneous and isotropic. Homogeneity means that the universe has the same composition and structure everywhere, while isotropy means it looks the same in all directions. This foundational assumption underpins much of the theoretical framework used by astronomers and physicists to model the cosmos.
Why does this principle matter? The significance of the Cosmological Principle is profound because it allows scientists to generalize observations made from Earth to the rest of the universe. If the universe is isotropic and homogeneous, then our local measurements can be applied universally, offering a cohesive picture of cosmic evolution. This principle makes it possible to describe the universe with relatively simple equations, such as those found in Einstein’s General Theory of Relativity.
One of the earliest confirmations of isotropy came from observations of the cosmic microwave background (CMB) radiation. Detected in the 1960s and later mapped in exquisite detail by missions such as COBE, WMAP, and Planck, the CMB shows remarkable uniformity in temperature, with only slight fluctuations. These fluctuations are essential—they are the seeds from which galaxies and larger structures grew—but their smallness supports the idea that the early universe was almost perfectly uniform.
Homogeneity, on the other hand, is harder to prove observationally because we can only observe a finite volume of the universe. Yet, large-scale surveys of galaxies suggest that the distribution of matter becomes more uniform the farther out we look. Projects such as the Sloan Digital Sky Survey (SDSS) have mapped millions of galaxies and demonstrated that, beyond local irregularities, the universe does appear statistically homogeneous.
Of course, the Cosmological Principle is an approximation. On smaller scales, the universe is clearly clumpy: stars gather into galaxies, galaxies form clusters, and clusters group into superclusters. But when these structures are averaged over hundreds of millions of light-years, the overall picture smooths out. It’s like viewing the ocean from space—it appears smooth, even though we know it is filled with waves and currents up close.
Some cosmologists have challenged the universality of this principle. Could our location in the universe be special in some way? This idea, known as the anthropic principle, suggests that our place in the universe may be uniquely suited to support life and observation. However, most evidence still supports the Cosmological Principle as a valid and useful approximation.
The implications of this principle extend far and wide. It influences how we model the expansion of the universe, calculate the age of the cosmos, estimate the density of matter and energy, and understand the ultimate fate of everything. Without the Cosmological Principle, our current cosmological models would be far more complex and less predictive.
In summary, the Cosmological Principle is more than a philosophical stance; it is a practical and empirically supported framework that enables us to understand the universe in a coherent way. It serves as a guiding star in the vast sea of cosmological inquiry, providing structure to our models and confidence in our interpretations.
The Unobservable Universe: What Lies Beyond?
If the observable universe defines the boundary of what we can detect, then the unobservable universe is everything that lies beyond that boundary. This cosmic frontier includes all regions of space from which light has not yet had time to reach us since the beginning of the universe. Because the universe is only about 13.8 billion years old, and because light travels at a finite speed, there is a horizon beyond which we simply cannot see—not now, and perhaps not ever.
But the existence of the unobservable universe is not just a logical conclusion; it also stems from the physical principles of cosmology. Due to the expansion of the universe—an expansion that is accelerating because of dark energy—some parts of the cosmos are moving away from us faster than the speed of light. These regions are effectively receding beyond our causal reach. No signal from them will ever reach us, and no probe we send out will ever reach them.
Astrophysicists often describe this as a “cosmic event horizon,” analogous to the event horizon of a black hole. Just as no information can escape a black hole’s event horizon, no information can enter our observable universe from beyond the cosmic event horizon. This challenges our notions of completeness and totality in scientific understanding. We are studying a universe that may be only a tiny fraction of a far larger whole.
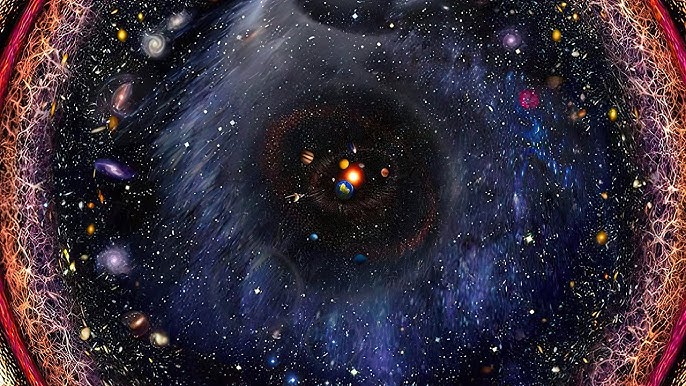
So, what might this unobservable universe look like? The truth is, we don’t know. However, if we assume the Cosmological Principle holds beyond our observational limits, we can infer that the unobservable universe is probably similar in structure and composition to what we can see. This is a key assumption, and while it is the most parsimonious, it is not provable. If, on the other hand, the Cosmological Principle breaks down on ultra-large scales, the unobservable universe might harbor phenomena vastly different from anything we can imagine—alternate laws of physics, different distributions of matter, or even entirely separate physical constants.
Quantum theory also suggests the possibility of a vast multiverse, in which our universe is just one bubble in a frothy sea of cosmic expansion. These other universes would exist beyond our observational capabilities not just in space, but potentially in entirely separate realms of existence. In this view, the unobservable universe is not merely more of the same, but something qualitatively different.
Even within the framework of our own universe, the existence of an unobservable region forces us to reckon with the limits of human knowledge. What does it mean to study the universe scientifically if we can only access a finite portion of it? Is the picture we form based on our observable patch truly representative of the whole? These are not just scientific questions; they touch on the philosophical foundations of epistemology and the nature of reality itself.
Technological advancements might push the boundaries of what we currently consider unobservable. For example, signals in forms other than electromagnetic radiation, such as gravitational waves or neutrinos, might allow us to indirectly infer what lies beyond. However, even these methods have practical and theoretical limits. Unless we develop entirely new physics or means of exploration, vast regions of the universe will remain permanently beyond our observational grasp.
The unobservable universe invites awe and humility. It reminds us that no matter how much we learn, there will always be more beyond the horizon. In a sense, the unobservable universe is the ultimate frontier—a vast, mysterious expanse that lies just out of reach, challenging our intellect and inspiring our imagination.
By contemplating the unobservable universe, we also gain perspective on the importance of our observational capabilities. Every photon captured by a telescope, every data point gathered by a space probe, becomes a crucial clue in assembling the most complete picture possible of the cosmos. And while we may never know what lies beyond, the pursuit itself is one of humanity’s most profound and inspiring endeavors
The Multiverse Theory
Among the most fascinating and speculative ideas to emerge from modern cosmology is the concept of the multiverse. The multiverse theory suggests that our universe may be just one of an immense, possibly infinite, number of other universes, each with its own unique properties, physical laws, and perhaps even dimensions of time and space.
This theory arises naturally from several different areas of theoretical physics. One of the most prominent is inflationary cosmology. According to this theory, a fraction of a second after the Big Bang, the universe underwent a rapid expansion known as cosmic inflation. During this process, quantum fluctuations could have caused different regions of space to stop inflating at different times, leading to the creation of “pocket universes” within a larger multiverse. Our observable universe would then be just one such pocket.
Another compelling line of reasoning comes from string theory. String theory attempts to unify all fundamental forces of nature, including gravity, into a single theoretical framework. One surprising implication of string theory is the existence of a vast landscape of possible vacuum states—each corresponding to a different universe with its own physical constants and particle types. In this view, the multiverse is not just a possibility but a statistical necessity.
Quantum mechanics also contributes to the multiverse discussion through the Many-Worlds Interpretation (MWI). According to MWI, every quantum event spawns new branches of reality, each representing a different outcome. In this model, there is a constantly multiplying set of parallel universes where every possible version of history plays out. Although not directly observable, this interpretation has gained traction for its elegant treatment of quantum indeterminacy.
While the multiverse is a tantalizing concept, it is not without controversy. One major challenge is its lack of direct testability. If other universes exist but are completely causally disconnected from ours, then we may never be able to detect or interact with them. This raises philosophical and epistemological questions about the nature of scientific theories: Can something be considered science if it cannot be tested or falsified?
Despite these concerns, some researchers are exploring indirect ways to probe the multiverse. One approach involves searching for anomalies in the cosmic microwave background that might be the result of collisions between bubble universes. While no definitive evidence has yet been found, advances in observational cosmology continue to push the boundaries of what we can infer about the early universe and its possible neighbors.
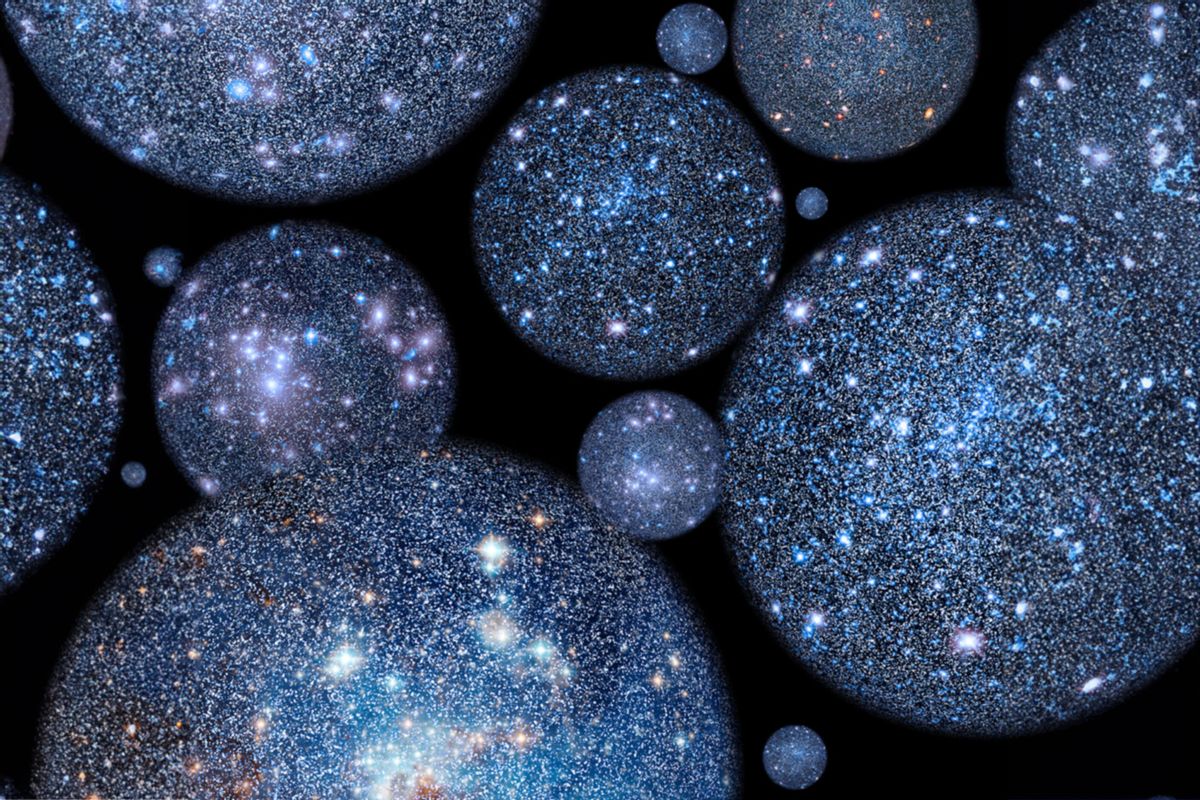
The implications of the multiverse theory are profound. If true, it would radically alter our understanding of the cosmos and our place within it. Instead of being the sole cradle of life in a finely-tuned universe, we would be one among countless possibilities, where life emerged by chance in a universe with just the right conditions.
The multiverse could also help resolve longstanding puzzles in physics, such as the fine-tuning problem. Why do the fundamental constants of nature seem so perfectly calibrated for the existence of life? In a multiverse scenario, these constants vary across universes, and we simply find ourselves in one where the conditions are right—because we couldn’t exist in any other.
Ultimately, the multiverse theory stretches the imagination and challenges the boundaries of science. Whether it is a physical reality or a mathematical abstraction, its exploration opens new avenues of thought about existence, reality, and the infinite possibilities that may lie beyond our cosmic horizon.
Observable vs. Unobservable Universe: A Matter of Perspective
The distinction between the observable and unobservable universe is largely a matter of perspective. From our vantage point on Earth, there are limits to what we can observe directly. However, as technology advances and our understanding of the universe evolves, these limits may change.
For instance, the discovery of cosmic microwave background radiation, the afterglow of the Big Bang, significantly expanded our understanding of the early universe. This discovery allowed us to observe parts of the universe that were previously unobservable.
Observation Techniques and Tools
The tools and techniques used to observe the universe are continually evolving. Telescopes, both ground-based and space-based, have allowed us to peer deeper into the universe. Other observational tools, such as neutrino detectors and gravitational wave observatories, provide new ways to gather information about the universe.
With the advent of powerful space telescopes like the Hubble Space Telescope, and soon the James Webb Space Telescope, our ability to observe distant galaxies and celestial bodies has significantly improved. These tools are crucial in expanding our observable universe and understanding the unobservable universe.
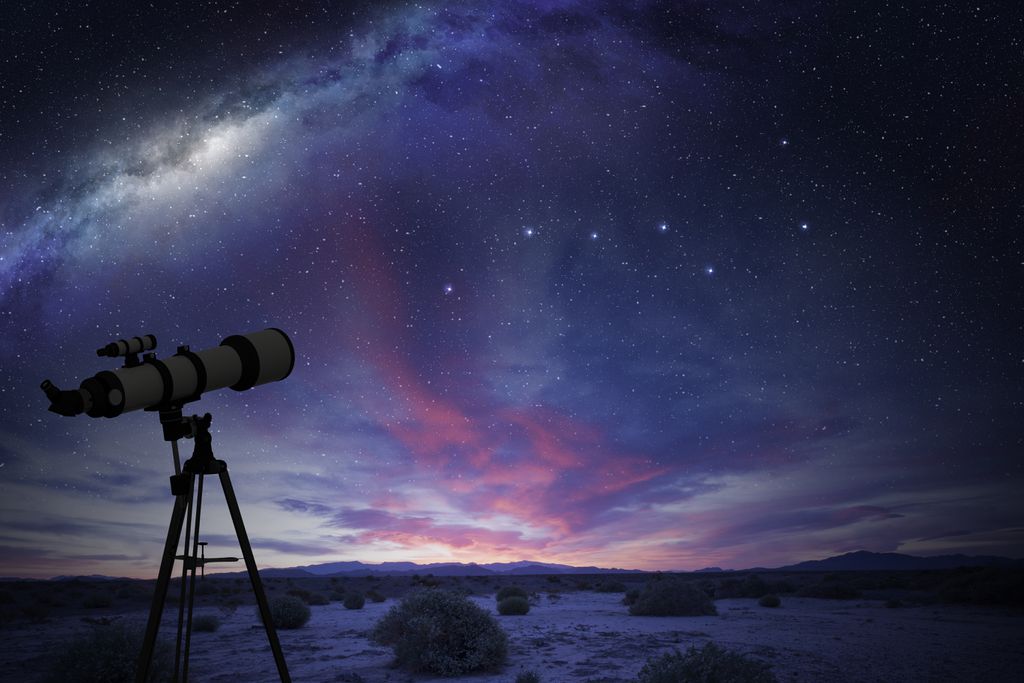
Conclusão
In conclusion, our exploration of the observable and unobservable universe has led us to discover profound aspects of our cosmos. The hidden depths of the universe, both observable and unobservable, continue to be a rich source of fascination and intrigue. By delving into these mysteries, we deepen our understanding of our place in the cosmos and the laws that govern it.
The universe, observable and beyond, presents us with a vast, complex, and ever-changing tapestry of galaxies, stars, and other celestial bodies. This intricate network, expanding beyond the reaches of our current technology and understanding, serves as a stark reminder of the grandeur of the cosmos and our ongoing quest to explore it.
Despite the challenges and limitations we face, advancements in technology and scientific theory continue to push the boundaries of our cosmic understanding. In doing so, we unlock new perspectives, leading to new theories, and possibly, new realities.
In the grand scheme of things, we are but a small part of an immense, mysterious universe – a universe that is as fascinating as it is vast. As we continue to look to the stars, we remain humbled by the beauty and complexity of the cosmos that surrounds us. A journey of discovery awaits us in the deep, uncharted territories of the universe. Let’s keep exploring, for the universe is not only about what we can see, but also about what remains unseen.